TITANS OF NUCLEAR
A podcast featuring interviews with experts across technology, industry, economics, policy and more.
Latest Episode

1) The beginning of Brian’s career and his time in the United States Navy as a diver, as well as what drew him to engineering and nuclear
2) Brian’s initial journey to Oregon State and all of the research projects he’s had a hand in since then
3) Fostering a passion for nuclear in the next generation of nuclear engineers and why the researchers as just as important as the research itself
4) What challenges and successes the nuclear industry will face in the coming years and how to form your individual opinion on nuclear energy

1) Neil’s background in the British Navy and his personal journey in the nuclear industry
2) The Institute of Nuclear Power Operations (INPO) and its work to increase a power plant’s capacity factor and uptime
3) EPRI’s research surrounding operations and maintenance cost as well as capital cost
4) Gen IV reactors and what we need to do now to support their implementation in the coming decades
Q1 - Entrance into Nuclear
Bret Kugelmass: Where were you born?
Neil Wilmshurst: Neil Wilmshurst was born in Plymouth, U.K., but grew up near Newcastle. While he was in school, Wilmshurst’s class took a trip to visit Calder Hall Nuclear Power Plant, the first generating plant in the world. Calder Hall was built to produce plutonium for the U.K.’s weapons systems. Wilmshurst’s father and grandfather were both in the Navy and Wilmshurst followed suit and joined the Navy to be a captain of a ship. He was selected early on to pursue an engineering degree in the stream to navigate fight ships. Wilmshurst ended up on a destroyer during the uprising in Beirut. From there, they went down through the Suez Canal and, during that trip, decided he wanted to be a submarine engineer. Four personnel were responsible for all the engineering aspects on the nuclear submarine. In the 1950’s, the U.K. decided it wanted a nuclear program, so Hyman Rickover allowed a technology transfer from the U.S. Navy to the Royal Navy, via Rolls-Royce Aero Engines. This technology was used to build the first U.K submarine, the HMS Dreadnought. After that, the two programs diverged and completed their own design work. The reactor is so critical and it must be robust enough to withstand the movement of the submarine and depth charges. Wilmshurst initially started at Sizewell B, a Westinghouse 4-loop SNUPPS plant, as a systems engineer for the diesels and cooling water systems. Wilmshurst was one of the first people the U.K. brought in from outside their nuclear program because of the new technology. Wilmshurst then became the in-service inspection (ISI) coordinator and moved on to run maintenance. In early 1998, British Energies started a partnership with PECO called AmerGen that was going to go buy nuclear plants and Wilmshurst joined on in mid-1998 as part of the team to do due diligence on Three Mile Island (TMI).
Q2 - Buying and Selling Three Mile Island
Bret Kugelmass: How did you find yourself in the financial side of nuclear?
Neil Wilmshurst: Along the way in the Navy, Neil Wilmshurst got plucked out of a job and sent off to do his MDA for a couple of years. AmerGen needed some technical people on their due diligence team to understand what it was they were thinking of buying with Three Mile Island (TMI). When considering purchasing a power plant, you look externally at the market, but also the condition of the plant, the staff, the training pipeline, the fuel contracts, the maintenance history, among other things. The value of the plant, warehouse, and decommissioning fund must be considered, as well as staff reduction funding and value of fuel in the reactor at the time of sale. AmerGen faced companies making the strategic decision to just enter the business, which would allow hits to the bottom line to purchase a plant and enter the business. AmerGen had the perspective there was a value there with tremendous revenue. The seller’s perspective was that it wasn’t their core business and they didn’t want to maintain the infrastructure and the risk. In 2003, Wilmshurst came over to the U.S. After the purchase of TMI, Wilmshurst was asked to stay there running work management. Because of some market issues in the U.K., where the U.K. changed the electricity market and futures prices of electricity plummeted. British Energy went bankrupt, went to the government for a bailout, and had to sell all its offshore assets, including TMI. Wilmshurst was ready for something different and joined the Electric Power Research Institute (EPRI) in the U.S. Wilmshurst was brought in to head up their equipment reliability efforts. Over time, the nuclear industry added more and more commitments, testing, and maintenance. The focus of equipment reliability program was to understand what needed to be done, how often it needed to be done, and making sure it was done right. Then, they had to systematically go through the designs of the plants and figure out the vulnerabilities that would create trips and downtime. When a reactor trips, the control rods may go in or you may lose a pump or transformer causing a power reduction.
Q3 - Probabilistic Risk Assessment in Nuclear
Bret Kugelmass: How did U.S. nuclear operations make a turnaround?
Neil Wilmshurst: A big part of the turnaround in U.S. nuclear operations had to do with probabilistic risk assessment (PRA), understanding the real risk profile of a plant. This allows people to go to online maintenance, rather than waiting for an outage. If you wait for a plant shutdown to maintain the safety equipment, the equipment may degrade and cause an unplanned trip if it fails. It many cases, it is safer to take a diesel out of service with a plant operating to repair a lower level outage than try and stagger on the outage and do the big repair. The Electric Power Research Institute (EPRI) is predominantly funded by the utilities, but also some funding from the U.S. government and global governments. EPRI is set up as a non-profit, requiring that they maintain their independence in serving the public good. The value of EPRI for their members is that they are independent and tell the story as it is, not the voice of the industry. The U.S. has 90 plus percent capacity in nuclear power, which became achieved by focusing on the outcomes and not so much the cost. The capital, operating, and maintenance costs are now in focus. There is still some overmaintaining and too much preventative maintenance on the plant, which requires many more parts; there are opportunities to optimize the maintenance. Your inspection scope for a plant can also be looked at from a risk-informed perspective to reduce the manhours. In 2010, Wilmshurst took the position of Chief Nuclear Officer at EPRI. Every career move Wilmshurst has taken has never been a zero risk strategy.
Q4 - Electric Power Research Institute
Bret Kugelmass: What are some of the areas that you saw back then that could use a fresh way of thinking and how has that translated over the last few years?
Neil Wilmshurst: Neil Wilmshurst inherited an organization which was very slow moving, tied to its past, and very U.S. centric. There was certainly a need for a greater global footprint and the time was right for some radical rethinking of the construct in how they interacted with their members. The way Electric Power Research Institute (EPRI) operated with their utility members is that they were happy to be in the room and not perceived as being on an equal footing with their members. Wilmshurst seeked to gain a level of trust in those relationships in which EPRI was no longer being viewed as another supplier, but were true partners with the utilities in their endeavors. This transformed into a lot more time on member sites and working with our members for the staff. About half of the staff in the nuclear sector at EPRI had worked at a nuclear plant. Wilmshurst aimed to get those that didn’t have that experience to the plants to understand their issues and challenges. EPRI also started an aggressive outreach outside the U.S. Of the global nuclear fleet of 400+ reactors, EPRI is interacting with over 90% of those plants; they fund EPRI’s programs. Nuclear plants used to view the fact that they were working with 1960’s technology, such as analog controls, as a positive because they were tested and reliable. Nuclear plants need to move into the digital age and embrace the digital effort and analytics. This will give benefits such as higher reliability and lower cost. EPRI also supports small modular reactors and Gen 4 reactors. In order to get to the Gen 4 reactors, you need to extend the life of the current fleet so that there is the infrastructure, staff, and supply chain to support the Gen 4 reactors.
Q5 - Connecting Global Nuclear Organizations
Bret Kugelmass: Is EPRI the vehicle carrying the nuclear industry into the future?
Neil Wilmshurst: In the U.K., a visionary called Lord Walter Marshall set up the World Association of Nuclear Operators (WANO). Marshall drove the nuclear industry in the U.K and in the U.S., Bill Lee set up the Institute of Nuclear Power Operations (INPO). The next group of global leaders is coming together and realizing that this is the time to rally the industry and get moving. The Nuclear Energy Institute (NEI) is talking about market construct and the national security and global impact of nuclear programs. The Electric Power Research Institute (EPRI) is working with the International Atomic Energy Agency (IAEA) and the Nuclear Energy Agency (NEA) of the OECD to look at what it takes to keep nuclear viable. They are working with a number of organizations towards a big conference in Korea next year around nuclear plant innovation and looking at innovations other industries have deployed to bring safety and economic benefits. The hurdle nuclear typically faces with deploying innovative technologies is the regulator. The Nuclear Regulatory Commission (NRC) is open to this perspective, supplier naturally see a commercial benefit, and utilities are starting to see technology as an opportunity to reduce costs. Getting global regulators more connected and more similar, it becomes easier for the supply chain. If you believe in climate change and look at where the energy market is going, there’s a growing consensus that, in order to achieve the CO2 reductions we need, there needs to be an 80% drop in CO2 generation. You cannot get there just by decarbonizing the electric industry, but also industry, transportation, commercial, domestic, and everything else. It’s important that the nuclear option is there and available to meet growing demand; to get there, the current fleet must be successful to drive the university courses, research infrastructure, and supply infrastructure.
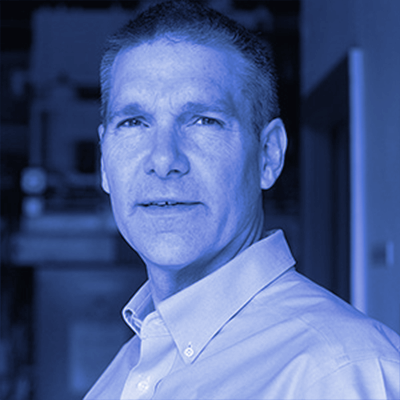
1) Eric’s personal evolution in the nuclear industry and his entrepreneurial endeavors including General
2) How a combination of cheap gas and lack of demand for electricity nearly eliminated the wind development industry
3) Commercialization development and deployment challenges with advanced reactors and how we can overcome them
4) Ways in which we can reduce the cost and time required in the construction process to increase nuclear’s scalability
Q1 - Early Exposure to Design
Bret Kugelmass: How did you become a climate change policy analyst and advisor?
Eric Ingersoll: Eric Ingersoll was born in London, lived there for five years, and then moved to Ghana with his parents. HIs dad was working on solving real problems in the world for people and Ingersoll saw this model as something he wanted to do. Ingersoll moved to the States in 1971 and found himself in a country filled with tension and alienation, compared to the exceptionally nice people he left in Ghana. When Ingersoll was in high school, his father was a solar architect doing passive solar and active solar thermal work, where Ingersoll learned how to do heat flow calculations for a building. He saw useful applications for his math classes and saw an experience, such as how a building feels, is amenable to some engineering and design. Ingersoll became interested in complex systems, leading him to design his own major in college. He also spent a lot of time as a child building and making things, taking responsibility for big projects, like re-roofing the barn, on his own. Ingersoll got a sense that you could teach yourself things and the mechanical and technical stuff is not out of reach. His mentality of thinking of things as design problems and used his formal education to fill in areas of knowledge that he thought would be useful.
Q2 - Meditation and Motivation
Bret Kugelmass: Did you start advising on policy right after University of California - Berkeley?
Eric Ingersoll: Eric Ingersoll has oscillated between entrepreneurial work and policy and advice work. During college, Ingersoll worked for a construction firm and hired on after graduation. At the time, he was very interested in the development of software for editing video. He grouped up with hardware and software grads from Berkeley, but the Avid software came out shortly after they started working together. Ingersoll got a taste for technology and technology start-ups, leading to work with other start-ups in the software and visual processing area. He started his own consulting firm, moved into a zen center, and was able to develop his motivation to benefit others. Ingersoll practiced meditation and began going on long meditation retreats, eventually staying at the retreat for silent meditation for one year. He spent months meditating on how he could help heal the planet and make it a better place. Around Earth Day in 1990, Ingersoll took his interest in energy and technical background and created the Green Pages, which gave a regional comprehensive listing specifically directed for environmental action.
Q3 - Environmental and Energy Startups
Bret Kugelmass: Tell me about harnessing human motivation towards accomplishing the goal of making our environment healthier.
Eric Ingersoll: Eric Ingersoll created the Green Pages, which gave a regional comprehensive listing specifically directed for environmental action. This was at a time in which companies were beginning to think about environmentally oriented products and corporate strategy where environment counts. Nobody had an environmental person on their staff. Ingersoll knew so much about the products and what mattered. He created a consulting firm, Environmental Advantage, with a group of recent MBA’s who were excited about using strategy as a way to create transformation in industries. They did a lot of work around sustainable forestry, but there was no market for the certified product. Environmental Advantage did a big project funded by multiple foundations looking at creating a forest products buyer’s group to get these vendors to buy the certified wood and create an industry. As he had success and his practice grew, Ingersoll wanted to do more stuff related to energy and saw that climate change wasn’t getting any better and economic growth was still strongly tied to carbon emissions. Ingersoll did a lot of work in the early days of the PV industry by making the case for scaling the solar industry and showing how you could use existing financial tools to make it work. After his work with the solar industry, Ingersoll moved to Boston and started a consulting firm playing a role as a turnaround guy for startups. Investors brought him in to redevelop the strategies for their startups. He joined one of his client startups, Mechanology, as the CEO and got interested in the idea of air as energy storage combined with wind energy. They created a subsidiary called General Compression and came up with a design that could provide 300 hours of energy storage.
Q4 - Wind Resources and Energy Storage
Bret Kugelmass: How did you use a product from a different industrial process for your energy storage?
Eric Ingersoll: A process called solution mining, which is dissolving a bottle shape in the salt, produces salt to be sold and the caverns are used to store natural gas. The company did a study of North America and showed how many caverns they could make in the locations where the really good wind resources were to be used for wind energy storage. General Compression got funding to develop and qualify their second stage compressor. While they were doing heavy duty technology development in Texas and building a demo facility in Texas, the company had incredible modeling tools. General Compression had qualified more than $5 billion of projects with the five leading wind developers. At that point, shale technology started to kick in and natural gas prices dropped. Until this point, utilities were pursuing wind technology, but the combination of gas prices and no growth in demand due to the economic crisis and within a year, all of their customers exited the industry. Ingersoll left General Compression in early 2013 and a couple years later they went out of business. After leaving General Compression, Ingersoll’s motivation to solve the climate problem was stronger. The U.S. has a lot of salt cavern potential, incredible wind resources, and lots of areas in the country without many people. Other countries are not as lucky in terms of renewable energy, so Ingersoll started looking for a global carbon emission problem and thinking about requirements for broadly deployable solutions to the energy problem. The solution needed to be cost competitive, adaptable to a wide range of markets, be manufactured rather than constructed, and produce zero carbon electricity, leading Ingersoll to advanced nuclear. Until this point, Ingersoll had been passively anti-nuclear.
Q5 - Manufacturing Advanced Reactors in a Shipyard
Bret Kugelmass: After setting up your constraints, did you find that advanced nuclear was one of the only solutions that would fit those constraints?
Eric Ingersoll: The two things that would fit those constraints are advanced nuclear and some kind of zero carbon liquid fuel. Eric Ingersoll downloaded all the papers from Oak Ridge National Lab (ORNL) and started reading into molten salt reactors and other advanced reactors. This led to learning about current Gen 3 reactors and realized they weren’t that bad, even though advanced nuclear may be needed to go into different markets. Ingersoll realized the current Generation 3 reactors were a possible candidate, but they had a different problem. Advanced reactors needed to be developed and brought to market which was a commercialization and development problem. The Gen 3 reactors had a deployment model challenge and were being delivered through a completely non-scalable model. These reactors require an experienced and skilled workforce, project management, and contractors to successfully do that. This can be done over time, but takes a long time to build those capabilities. Ingersoll came across a project at MIT which was shipyard manufacturing of floating nuclear power plants. Ingersoll joined the group and completed studies on how much the cost of a nuclear power plant could be reduced if it was built in a shipyard. If you built a twin APR-1400 in a shipyard and delivered it in one piece to the location where it was going to be used, they found they could deliver it for about $2,500 per kilowatt anywhere in the world. The shipbuilding industry has been innovating in an environment of tense price and cost competition where quality standards have to be high. Productivity is lower in construction, of which nuclear is on the low end, than productivity in manufacturing.
Q6 - Cost-effective Manufacturing
Bret Kugelmass: Does your company sell these manufacturing models for advanced reactors?
Eric Ingersoll: Eric Ingersoll’s group developed a lot of intellectual property (IP) to build these reactors in shipyards but were not able to raise money for the company. This project is a difficult sell to the traditional investor community. The Chinese and Russians have started working on small floating reactors, but are not cost-effective because they are designed for specialized applications. The Hyundai shipyard in Korea and produce a hundred large ships a year. These nuclear plants are more complicated than a ship, but are not more complicated than offshore gas processing platforms. The global industry could easily make 100 of these reactors a year. Right now there is a major downturn in the global shipbuilding industry, so there may be interest for this capacity. In Ingersoll’s consulting work, he does a lot of work on cost reduction for existing nuclear and gigawatt scale new nuclear builds. There has not been the same kind of intentional, broad approach for looking at cost reduction opportunities in the U.S. and the U.K. Nuclear plants started out more expensive in countries that are now lower cost. Those countries had a national imperative and concerted effort to reduce those costs. They looked at where there was waste, work that was not added value, production aspects that were taking too long, or steps that could be automated. Both China and Korea focused on reducing cost. They started out recognizing they were at level zero and needed to build out that capability. In the U.K. and the U.S., people had forgotten a lot of the basic nuclear stuff and there may have been a lack of humility in that they couldn’t say they needed to learn the technical knowledge again. The nuclear industry has not figured out how to integrated quality and production.
Q7 - Integrating Quality and Production in Nuclear
Bret Kugelmass: How do you make quality and production complement each other?
Eric Ingersoll: Nuclear components often cost three to ten times as much as regular components and it has to do with the nuclear quality requirements. These are not produced in a high enough volume that anybody has thought how to automate that or design a process in which it is integrated into production. There is a lot of room for automation in the construction process. Nuclear is getting more successful and getting R&D funding, but no money is going towards automation. One of the benefits of having a detailed plan and 4D simulation is that it could be shared with lots of people, so people on site can know what they are going to be doing. Hitachi was worried about the contractors they were going to be using in the U.K. and there was nervousness on the contractor side on how risky the project was going to be and how they would bid the project. Hitachi decided to run a workshop for a couple weeks rehearsing the assembly of the reactor building and utilities on the critical path. The contractors thought the risk was much lower after sitting through the workshop and were more willing to contemplate the idea of fixed price bids. The uncertainty of risk comes from not having a clear idea of what needs to be done. Detailed planning cannot be done without a complete design.
Q8 - Nuclear’s Place in Fighting Climate Change
Bret Kugelmass: Why is it important that we pursue nuclear?
Eric Ingersoll: There is an unprecedented challenge in trying to solve the climate energy problem. The problem is considerably worse, and has the potential to be much worse, than is commonly understood. We are only now beginning to think through the consequences of these things, because they are multifaceted and interdependent on various things happening. Sea level rise has two effects: having the sea level go up every year and affecting coastlines and erosion, and making storm surges higher. The more energy in the atmosphere from additional heating and sea temperatures means stronger storms. When those happen infrequently, they are challenging and difficult, but when they happen frequently, we may no longer be able to ensure large parts of the cities on the coast. This threshold could be reached in the next 15-20 years. We do need a lot more energy to bring the world out of energy and our society will not stop using energy. Large amounts of low-carbon energy will need to be produced and large amounts of carbon dioxide will need to be removed from the atmosphere. Large amounts of nuclear energy are needed to have a chance of eliminating the worst impacts and adapting to the impacts of this change. The entire liquid fuel system of the plant has to be replaced with zero carbon energy.
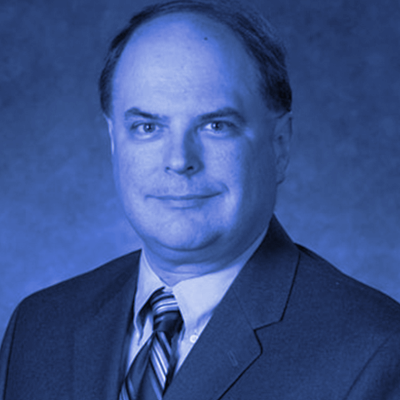
1) Gene’s background in nuclear engineering and personal journey in the nuclear industry
2) The history of nuclear in the US including the aftermath of the Three Mile Island accident
3) Economic challenges that impeded the nuclear renaissance
4) Why the market doesn’t yet recognize those benefits and how today’s oversupply of gas slows down the push for nuclear energy
Q1 - Entrance into Nuclear Engineering
Bret Kugelmass: How did you decide to pursue nuclear engineering?
Gene Grecheck: Gene Grecheck’s father, an immigrant from Eastern Europe after World War II, was an electrical engineer. As a child, Grecheck was always taking things apart and trying to figure out things worked. Grecheck attended Rennselaer Polytechnic Institute, starting out in physics. He decided he didn’t want to be a physicist, but was interested in energy and was bothered by the fact that most electricity in the U.S. was generated by burning stuff. At this time, in the early 70’s, the first commercial nuclear plants were going online in the U.S. Grecheck completed his Bachelor’s of Science in physics and stayed to complete his Master’s in nuclear engineering. After Grecheck started working at Virginia Electric and Power Company, which later became Dominion Power, Grecheck began taking night classes for his MBA at Virginia Commonwealth University. Grecheck saw nuclear technology as a piece of the big picture to make nuclear successful, which fed into the early 80’s focus on not building new plants, but making existing plants work after Three Mile Island. While at Virginia EP&C, Grecheck started out in the licensing department which was responsible for getting license applications approved by the Nuclear Regulatory Commission (NRC). The company had two operating units just started up at Surry and North Anna 1 and 2 were finishing up in construction working towards an operating license. At this time, there were four more units with construction permits issued, two each at Surry and North Anna. Grecheck’s first focus was assisting the lead engineer on North Anna 1 and 2. He read the Final Analysis Safety Report for this application from beginning to end.
Q2 - Nuclear Reactor Operators
Bret Kugelmass: Did starting out in licensing give you familiarity with every system in the power plant and enable your rise to site vice-presidency at Surry?
Gene Grecheck: Licensing seems like a detour off the engineering path, but that is one of the few places where you are expected to know a little bit about everything in the plant. There is very little specialization at that point, and you must be able to understand how everything fits together and where the experts are. Gene Grecheck found licensing an extremely good background. After receiving the license for North Anna, Grecheck was sent to Surry to get a senior reactor operator (SRO) license. In order to operate the controls of a nuclear power plant, the individual has to have an individual license from the Nuclear Regulatory Commission (NRC), which is achieved by passing a comprehensive exam that is administered by the NRC. Dominion made it clear that, if you wanted to rise in management of a power plant, you must get your SRO to make sure you understood how everything fit together and were not just focused on one thing. Some things an SRO must remember include the mark numbers of several hundred different components and their sequences and plant emergency procedures. Individuals were also evaluated on performance in the simulator, a computer-driven replica of the control room which can cause anything to fail. In the early 80’s, when Grecheck achieved his SRO, there was a lot of reactiveness to problems and not much time for management to get involved. The early reactors were designed without computers and calculators. Many of the assumptions and insights were not documented or turned over to the operator, even though they were input into the design. One by one, plants in the 1980’s and early 1990’s were discovering that the plants were not quite designed right, mostly because something had changed after the original design. At Surry, some instrumentation was added in response to Three Mile Island, but discovered the heat load within the switchgear room now exceeded what the ventilation system was designed to handle. The original heat load had not been documented anywhere.
Q3 - Human Factors in a Nuclear Power Plant
Bret Kugelmass: How do little things cause big problems down the line in nuclear reactors that are difficult to anticipate?
Gene Grecheck: Westinghouse and GE had the structure in place to learn some of those lessons the first time around. When Westinghouse was designing the AP-1000 and GE was designing the ESPWR, there was a lot of discussion of documenting the assumptions and margin management. If the designer knows there is margin in the calculation, it needed to be explicitly identified so someone changing it later on would not have to guess or recreate it. In the early 2000’s, Gene Grecheck was encouraged when the new designs were coming out because there was recognition that plants couldn’t be built that we don’t know how they work or have lost margin. The Generation 3+ design process incorporated lessons learned and some really good plants could have been built, but we got caught in the fact that those plants were not economic anymore. In the early 80’s, typical industry capacity factors were in the 60% range. This means that 40% of the time, the plant was not running due to trips and refueling outages scheduled for 90 days. Outages were major management challenges because there were always unexpected things found. Routinely, outages ran a minimum of 60 hour weeks for 70-90 days and the industry discovered that when people worked that much, judgment was impaired, people were not getting enough sleep, and were having trouble at home with their families. The nuclear industry created an incredible opportunity to boost the knowledge in many disciplines that had nothing to do with nuclear, such as how to improve human performance and identify error-likely situations. Human factors in the early days was focused on the operator interface in the control room and how to organize the displays to lead the operator’s eye to the right place. In an emergency situation when you’re not thinking clearly and facing a situation you haven’t seen before, you can help the human by focusing the limited attention span of people on the right stuff. Human factors did not only apply to operators, but also maintenance, engineering, and management. Some sites with multiple units are mirror images and some are copies. On the equipment side, an incredible amount of work went into single point failures.
Q4 - Nuclear Reactor Management
Bret Kugelmass: Tell me about single point failures.
Gene Grecheck: In the early days, the regulatory structure was focused on redundancy for safety function, meaning designing against the worst possible accident. The worst possible accident was a double-ended break of a main reactor coolant pipe (LOCA), which would cause you to lose all the fluid in the reactor coolant system very quickly and the plant had to respond. Redundant equipment was provided so you could survive the worst single failure. Typically, the worst single failure was a loss of electrical power, so there were two totally independent trains of electrical power and redundant pumps would be on independent sources of electricity. There was not recognition early on in design that a lot of these events could be prevented if you didn’t cause transience in the first place. Reactor trips were accepted, but that perspective began to change. By the 2000’s, there were multiple reactor operators that would train in the simulator for what to do in a reactor trip and work up to nine years without seeing the reactor trip. One of the things the Institute of Nuclear Power Operations (INPO) was created to do was realize - pre-Three Mile Island - that there was not a lot of recognition about the specialness of nuclear jobs. Most of the utilities that had built nuclear plants had built and operated coal plants, and nuclear was a snazzy new way of producing electricity but not fundamentally different. In the early days, management structures at nuclear plants were modeled after coal plants, meaning engineering was not plugged into the operations and the plant manager reported back to corporate at a low level. After Three Mile Island, people started realizing the different skill sets required. There were no lessons learned across nuclear plants in the early days. INPO created opportunities for people to get together, such as a senior plant management course. Dominion had corporate functions that made sure stations were operating properly. After realizing they had lost design control, they made a strategic decision that engineering was so important that it needed to be able to establish design authority, a technical ownership of the design of the plant. In order to maintain that division, the top engineering person at the station reported off-site to a corporate engineering structure. As Dominion began to expand and buy plants out-of-state, it became more and more important to have the corporate structure. After Dominion bought the Millstone plant, Gene Grecheck worked on loan to Northeast Utilities during the transition, acting as the VP of Operations for the plant.
Q5 - Changing Nuclear Plant Ownership
Bret Kugelmass: Is it tough for the staff of a plant to switch ownership, change the way they’ve been doing things, and see new leadership come into play?
Gene Grecheck: In the early 2000’s, many of the plants that were being auctioned or sold were already not economic. For many of the people in the plants, the plant either gets sold to a fleet operator or it shuts down. They were faced with a situation in which people had worked at the plant for 25-30 years and were used to a particular company culture. For the most part, people welcomed the buyer, since they were keeping them employed and making an investment in the employees and the plant. There were a lot of career opportunities opened up when a large fleet operator took over. The culture at Millstone was very different than what Gene Grecheck was used to. It took many years and many different Dominion leaders to integrate the plant into the Dominion system. Millstone had a history of lots of excess resources being spent on the process. By this time, Grecheck had spent 5-7 year as a site VP at Millstone and was ready for something different, just at the time of the nuclear renaissance and the company was starting to think about doing something with new nuclear. The Department of Energy (DOE) had a new program called NP2010 and had a number of opportunities to do some cost sharing if you could come up with proposals for site evaluation or technology evaluation. Under Grecheck’s leadership, Dominion was the only company to participate in every phase of NP2010. From a personal standpoint, this changed Grecheck’s life as his focus shifted from internal operations to external policy, federal and state agencies, and the public.
Q6 - U.S. Electricity Demand and Power Sources
Bret Kugelmass: What were some of the economic challenges that prevented the next level of growth anticipated during the nuclear renaissance?
Gene Grecheck: When the nuclear renaissance started with the adoption of the Energy Policy Act of 2005, the Department of Energy (DOE) had NP2010 and the Nuclear Regulatory Commision (NRC) had geared up to be able to issue licenses under the new Part 52 combined license process. Under the legislation, there were loan guarantees available and other incentives, resulting in a major storm of people falling over each other to submit applications for Westinghouse, GE, Mitsubishi, and other technologies. Gene Grecheck showed people in public presentation that the growth of electricity demand was going to double. If it was not going to be clean generation, it was going to be coal, and people were supportive of nuclear. The markets were a combination of traditional regulated utilities and some merchant plants. In 2007, natural gas prices started to drop and the recession hit the U.S, causing electricity demand to go away. Some places in the U.S. had negative electricity demand growth. As time went on, the markets were getting even further distorted by out-of-market incentives provided for renewables that were subsidizing them to the expense of something else. Some utilities had “must take” requirements, where they had to take all the renewables offered to them regardless of the cost. The application for North Anna 3 was completed in 2007 and Dominion recognized that the plant would not be built in a merchant situation, but instead a more conventional cost-of-service situation. By the time Grecheck retired from Dominion in 2013, Dominion received a combined license (COL) for North Anna 3, but it was put on a shelf. The fundamental issue is that, from a purely economic standpoint and with gas prices what they are, there is nothing you can do in the big nuclear space to make current technologies economical. Nuclear power is clean energy and does not produce the typical pollutants that come from burning things, such as particulates and nitrous oxides. There are approximately two billion people that have no regular access to electricity, limiting access to things like modern medicine and water. Solar and wind are not effective in these environments, so coal is the go-to power source.
Q7 - Gaps in Nuclear Policy
Bret Kugelmass: Why isn’t bringing the world out of energy poverty with clean energy the next “space race” challenge?
Gene Grecheck: The reason NASA was created and astronauts were sent to the moon because the U.S. and the U.S.S.R. were locked into a cold war or a struggle and everyone believed space was the next frontier. If you didn’t show superiority in space, you were demonstrating that you were technologically behind and it was very geopolitical. In the years since, NASA has been able to demonstrate that the spin-offs and the benefits to the U.S. economy and the world are tremendous from the technologies that were developed for the space program. If there could be a consensus around the idea that energy was important and if we could get consensus around the idea that if we’re going to produce energy, it should be done in the least environmentally impactful way, it stands to reason that nuclear could stand. But there is no consensus around the need to restrict carbon and no consensus on the need for adequate energy. Rather than coming up with the new technologies necessary, we are allowing perfectly good, seasoned, operating plants - that have been generating clean electricity for 40 or 50 years - to be shut down. Every time one of these plants are shut down, it’s not being replaced with solar or wind, it’s replaced with gas. In Gene Grecheck’s role as president at the American Nuclear Society (ANS), they created a committee that was going to work with the states to help understand policy implications because they thought Obama had just issued a clean power plan. They found that, at the state level, many of the policy makers had very little understanding of the economic impact of the nuclear plants, the number of jobs connected to the plant, and how much clean energy would be generated. Political rivalries exist between alternate energy technologies and if you are in the business of producing fossil fuels, you are not going to be supporting economic policies that promote nuclear.
Q8 - Telling the Nuclear Story
Bret Kugelmass: What topics do you help others with through your consulting practice?
Gene Grecheck: Gene Grecheck is a true believer that nuclear technology is not only desirable, but necessary. Grecheck helps people navigate their way through the thicket of how to go from a really cool idea to something that could be built. Most entrepreneurs in the nuclear sector don’t really understand the licensing process and what it means to go to the Nuclear Regulatory Commission (NRC) and get an application approved. Grecheck also helps others understand the economic factors of nuclear technology. There have been a lot of lessons learned over the years about how to manage these enterprises and how to coordinate design with operations. There are other facilities that deal with technology that have many of the same issues, such as how to make a quality assurance program effective. Just about everything we do today depends on energy and electricity. The future will have more dependence on power to support a transportation industry that is moving towards electric. It doesn’t make sense to depend on finite quantities of fossil fuels. Nuclear power is a way to do it in scale without covering the landscape in machinery that will not last that long. For way too long, the story has been told by people who will conflate the peaceful use of nuclear energy with weapons. Nuclear is the break in the world and the industry needs to be innovative. Nuclear needs to get actively engaged in setting the narrative. The American Nuclear Society (ANS) is an important part of a nuclear professional’s life. It is more than networking, but also developing the ability to communicate. Organizations like Generation Atomic are looking at ways to engage grassroots people to tell the nuclear story outside the traditional media. These organizations have the potential to move us from just talking about technical stuff to why nuclear is important and why the future of humanity depends on it.
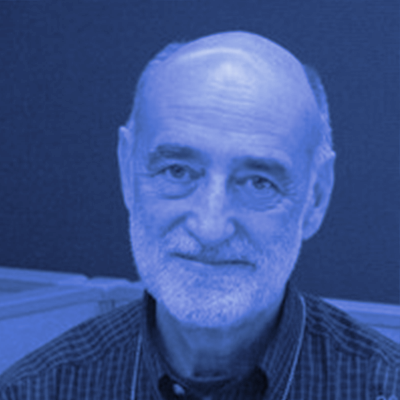
1) Richard’s background in physics and engineering and his personal journey in the nuclear industry
2) A deep dive into the Fast Breeder Reactor Program sponsored by the Department of Energy
3) How the NRC interacts with developers to bring forth new technologies including the AP600
4) Richard’s recent work with AdSTM providing consulting and training to regulatory bodies including the NRC
Q1 - Early Projects at Los Alamos National Lab
Bret Kugelmass: How did you get into engineering and physics?
Richard Barrett: Richard Barrett started out looking at looking at an academic career with a PhD in physics from the University of Virginia. When it came time to complete his postdoctoral work, Barrett saw more opportunities in applied research and ended up going to the Los Alamos National Laboratory. There were about 7,000 people at Los Alamos at the time. Barrett’s job was to write and apply computer codes for the specific purpose of doing calculation of the way in which neutrons interact with devices such as nuclear fusion reactors. The cross-section is a metaphor for how big the nucleus looks to a neutron that is approaching it. Neutrons behave differently in different energy groups, but with today’s technology, there can be many more groups and the way these cross-sections are used can be fine-tuned. A group is a range of energies reflecting a weighted average. Barrett’s coding at Los Alamos was for development of the fast breeder reactor program, which was sponsored by the Department of Energy (DOE) during the Carter administration. In 1978, Barrett also became involved in a group that was developing a visual model of the 1975 United States energy system. This data of where energy was being found, mined, stored, converted, and used was kept by federal agencies, state and local agencies, universities, and corporations. These spaghetti charts broke out the use of energy by sectors - industrial, commercial, residential, and transportation - and within each sector, how much energy was useful and how much energy was lost.
Q2 - Nuclear Reactor Safety Systems
Bret Kugelmass: How did you transition over to the Nuclear Regulatory Commission?
Richard Barrett: During Richard Barrett’s last two years at Los Alamos National Lab, Barrett was on loan to the Department of Energy (DOE) headquarters in Germantown, Maryland. He worked with the Office of Energy Research completing assessments of the quality and usefulness of their research programs. Around that time, the Reagan administration came in and they had a different idea of how the U.S. should be dealing with energy, with a stronger focus on industry over government. Barrett was impressed with the quality of people and the clarity of the mission at the Nuclear Regulatory Commission (NRC), leading him to accept an offer for employment. He started out working in the Office of Nuclear Reactor Regulation, where he spent most of his career, which deals with the direct interaction with the reactors. The NRC was most concerned about the reactor safety systems. The frontline safety systems are those which are meant to shutdown the chain reaction if anything goes wrong, so you are not dealing with the power and nowhere to put it. Systems must also be in place to replenish the emergency core cooling systems if there is a coolant leak. Other systems include heat removal systems, and backup systems, which support frontline systems. These include electricity, compressed air, and batteries, among others. Systems must be designed to meet the requirements during normal operation and also robust enough and fast enough to meet the demands during an accident. Design basis accidents or events involve failures of the reactor systems. The purpose of a design basis event is to check the capability of the system. A beyond design basis accident, such as a station blackout, is looked at in a probabilistic way.
Q3 - NRC Reactor Design Guidelines
Bret Kugelmass: Is there a catalog of all the things that could possibly happen at a nuclear reactor?
Richard Barrett: There is a catalog of all the things you have to look at in nuclear reactor design, not just accidents. The Standard Review Plan has 19 chapters, such as electrical, instrumentation, and materials, which contain sections and subsections totalling about 300 topics. This document provides guidance on what has to be submitted, qualifications for people who are reviewing it, criteria for acceptability, technical guidance available, and other details. Regulatory Guides go into detail about specific topics, such as the assessment of the possibility that there will be an explosion of a munitions factory in the vicinity of the plant, or a train full of munitions that goes down the tracks a few miles from the plant. There is a Regulatory Guide that tells you how to assess the likelihood of it and the consequences in terms of pressure exerted on the plant. Richard Barrett became part of the Senior Executive Leadership Service, a group of executives there to provide continuity when there is a change in administration. There was a three year period in which Richard Barrett was responsible for the licensing of thirteen nuclear power plants in the Midwest.
Q4 - Reactor Design Applications to the NRC
Bret Kugelmass: What advanced reactors were being designed in the 1980’s?
Richard Barrett: In the 1980’s, advanced light water reactors designs were being pursued. A boiling water reactor design and a pressurized water design were approved. A passive design called the AP-600, which eventually became the AP-1000, got certification and two plants based on that design are currently under construction. A passive plant has safety systems that do not rely on electric power, but instead passive mechanisms such as in the form of batteries, or gravity. The AP-1000 reactor is designed so that the frontline safety systems can operate in the absence of AC electric power. This review involved capabilities of systems that haven’t been used before and computer codes which have been validated through testing on scale model types of reactors. The Nuclear Regulatory Commission (NRC) encourages designers to come in early and have pre-application discussions to focus on what’s new and different in the design. NuScale’s design cools the reactor without reactor coolant pumps. The NRC would want to have early conversations with NuScale to find out what the technical basis is that can justify it can work reliably and with margin. The NRC started to develop a process to communicate with applicants and determine what their plans were.
Q5 - Risk in Nuclear Design and Operations
Bret Kugelmass: What is a risk informed application process?
Richard Barrett: A risk informed process is not risk based; criteria is not set aside. Risk informed application takes into account the consequences of the accidents that are involved without getting rid of defense in depth, margin, and risk numbers. The allowed outage times that could be taken for some safety equipment was changed based on an acceptable block of risk. For three years, Richard Barrett was in charge of the agency’s emergency response program. There was an emergency operations centers at headquarters and regional offices with operations officers on duty 24/7/365. This group was in contact with all utilities, major facilities, federal agencies responsible for emergency response, and state and local governments responsible for protective actions such as evacuations and sheltering of people. The ability of motor operated valves to open and close to meet their design requirements became a big problem, as they would become locked because of the differential pressure across the valve stem. In one case, a tornado did a 360 degree circle around a plant and took out all the off-site power. You have to be ready for things that can’t happen, because they do. At the NRC, a very high priority is placed on gathering operational experience, which is analyzed for trends and patterns.
Q6 - Role of NRC Globally
Bret Kugelmass: How is transparency at the Nuclear Regulatory Commission used as a positive?
Richard Barrett: If the information is put out there, you help people understand what it means, and the public has an opportunity to speak, transparency builds trust. Worldwide, transparency is one of the things that makes the Nuclear Regulatory Commission (NRC) a hallmark, since you can find out what they are thinking in real time and how and why they are reacting to what’s happening. During Fukushima, the NRC was there with their expertise to help them think through it, as well as at Chernobyl. The NRC participates in IAEA (International Atomic Energy Agency) programs and the NRC participates in important events around the world. The NRC wants to know how their plants will be affected, but also to provide assistance to the host country. In the risk informed area, there was a proposal for rulemaking that would have made more use of risk in terms of the way in which components in systems have to be designed. Nuclear grade systems are extremely expensive compared to other industries, since they have to meet environmental qualification requirements, deal with external events, and meet certain quality assurance requirements. This risk informed approach would allow safety systems to not be treated as “gold plated”. This proposal was eventually passed, but there was a great deal of disagreement. Over the last ten years, Richard Barrett has been working with ADSDM, who has a contract with the NRC to provide consulting and training to regulatory bodies in countries that are emerging nuclear power countries.
Q7 - Future Nuclear Development
Bret Kugelmass: What is an example of something that might catch an emerging nuclear power country off guard?
Richard Barrett: One big surprise for emerging nuclear power countries was that it wasn’t so important to train a bunch of nuclear physicists, contrary to having really good nuclear grade welders. If you don’t have the infrastructure, it is difficult to build and operate the nuclear stations. The International Atomic Energy Agency (IAEA) started the Milestones program, which was defined to assist an emerging nuclear country in the decision about whether they should go into nuclear. If they were to go into nuclear, IAEA helped teach them what kind of people, tools, computer codes, infrastructure, and financing they would need. It’s easy to look at the nuclear industry in the U.S. and focus on the nuclear power plants that are shutting down and saying it could be the end of nuclear power in the U.S. Richard Barrett is inspired by motivated people forming new companies in industry and excitement at the National Labs to support these companies. The NRC is being innovative and environmental people are supporting nuclear.
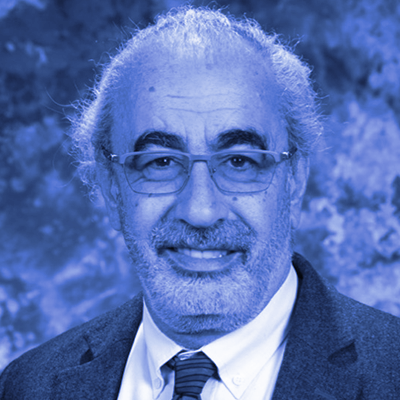
1) Kemal’s background in thermodynamics and personal evolution in the nuclear industry from Los Alamos to Idaho National Labs
2) Idaho National Lab’s GAIN (Gateway for Accelerated Innovation in Nuclear)
3) Kemal’s focused role on a new test reactor and its significance in research and development in nuclear technology
4) Building support for nuclear in US Congress
Q1 - Nuclear as an Energy Solution
Bret Kugelmass: Where did your interest in nuclear begin?
Kemal Pasamehmetoglu: Kemal Pasamehmetoglu’s interest in general about issues related to energy and energy for society started when he was in high school. Pasamehmetoglu grew up in Istanbul, Turkey. The city that he was in was growing too fast and there were a lot of infrastructure issues; electricity was not readily available. People who never had electricity get used to it, but those who had it for a while and had it taken away from them, got frustrated. Rolling blackouts were not common until the late 60’s and early 70’s, but all of a sudden it started happening. Pasamehmetoglu decided that he wanted to be an engineer and that was the problem he wanted to tackle: how to provide affordable, clean, accessible energy to people. Pasamehmetoglu studied mechanical engineering, with a focus on thermodynamics of energy systems, at Boğaziçi University, where he took a couple nuclear engineering courses. After his undergraduate, Pasamehmetoglu came to Florida to complete his Master’s and PhD. His first research project in the early 80’s was on the solar energy sector, convincing himself that, as good as it was, solar wasn’t going to work. His PhD research and dissertation was on nuclear reactors, looking at how to mitigate loss-of-cooling accidents (LOCA) at a time shortly after the Three Mile Island incident. A large-break LOCA is when you get a guillotine break in the pipe and the pressure quickly pushes the coolant out. The pipe could also start leaking, which takes a longer period of time for the reactor to depressurize and lose coolant. Slow leaks are not as catastrophic, but it puts the operators in a position where they don’t know what to do.
Q2 - Projects at Los Alamos
Bret Kugelmass: When you lose your coolant in the reactor vessel, do you lose the moderator and what happens to the reactor?
Kemal Pasamehmetoglu: When the reactor vessel loses pressure in the coolant, the reactor shuts down and decay heat remains. The decay heat can still heat up the remaining fuel in minutes. One thing in analysis is the point at which the liquid left in the system can no longer cool the fuel rods, which is called critical heat flux. Kemal Pasamehmetoglu went to Los Alamos to continue on his research, where they were currently developing the code that the Nuclear Regulatory Commission (NRC) currently uses to model accident scenarios. Pasamehmetoglu spent twenty years at Los Alamos. In the late 80’s, Hanford had an advanced waste process that was producing hydrogen, and everybody was worried there would be a hydrogen explosion in the tank. This was declared the highest safety priority for the Department of Energy (DOE) system. Pasamehmetoglu worked on putting a pump inside the 1 million gallon waste tank, stir the waste continuously to avoid accumulation of hydrogen, and its release. The Belgians are working on a project called MYRRHA, a subcritical accelerator driven system that takes actinides and reduces them, creating energy in the process. Excess neutrons come from the spallation process of irradiating heavy metals with protons. Pasamehmetoglu worked as project manager on the U.S. equivalent of that project, called accelerated transportation of waste. It is not an easy or cheap technology, since it consumes a lot of energy itself. Because it is subcritical, you don’t have to worry about your feedback effects of fuel.
Q3 - Fuel Research at Idaho National Labs
Bret Kugelmass: How long were you at Los Alamos?
Kemal Pasamehmetoglu: Kemal Pasamehmetoglu worked at Los Alamos until 2004, when he was working on the extension of the accelerated transportation of waste project, which had become a fuel cycle project. At this time, the Department of Energy (DOE) decided they were going to create the Idaho National Laboratory (INL). The head of the DOE at the time, Bill Magwood, asked Pasamehmetoglu to go to INL, which was established by February 2005. Pasamehmetoglu became the division director for nuclear fuels and materials. Researchers work on fuel are trying to better understand and characterize existing fuels, develop similar fuels to be more accident tolerant, develop advanced reactor fuels. Pasamehmetoglu was also the National Technical Director for all the DOE related fuel research. The focus was not necessarily on accident tolerance, but instead high burnout, reduced waste, and overall higher performance light water reactor fuels at a lower cost. Fukushima happened during Pasamehmetoglu’s work on this program, shifting focus onto accident tolerance. Pasamehmetoglu became the Associate Lab Director for Nuclear Science and Technology, where he spent five years during which he gave multiple presentations to the DOE about a program similar to GAIN, which helps innovation in the nuclear space by making facilities and expertise more available.
Q4 - Nuclear Test Reactors
Bret Kugelmass: As Associate Lab Director of Nuclear Science and Technology, what conversations did you have with your team about what could be done to launch the nuclear industry forward?
Kemal Pasamehmetoglu: There are a lot of choices in terms of advanced reactors that can solve or mitigate problems. As many choices as there are, there are as many proponents and opponents of those choices. One of the mistakes made in the past was letting national laboratories and the government decide what is good and bad, instead of letting the industry take the lead with assistance and technical advice. Doing research for the sake of research was not necessarily the right thing for Idaho National Labs (INL) to do, thinking that they had the answers and nobody else did. Gateway for Accelerated Innovation in Nuclear (GAIN) was established when Pasamehmetoglu was still associate director, but he served as the director of GAIN for the first several months to get it started. Pasamehmetoglu brought Dr. Rita Baranwel to INL where she took over GAIN as director. A year ago, Pasamehmetoglu took a job as the director of the test reactor at INL. We currently don’t have access to large quantities of fast neutrons to do fuels and materials testing for the advanced reactors. Materials behave differently dependent on the neutron energies. Fast reactors operate at a very high flux, which is neutrons per centimeter squared per second. Peak neutron flux in a light water reactor is on the order of 10^13 neutrons per centimeter squared per second, but in fast reactors, the order is 10^15. The only fast reactor left, when all the companies were coming along try to complete designs to get to commercial market, was in Russia. The communited decided to look at what it would take for the U.S. to regain that capability.
Q5 - Government Support for GAIN
Bret Kugelmass: How do you turn a test reactor from a conversation topic in the nuclear community to a project with government support?
Kemal Pasamehmetoglu: The Department of Energy (DOE) has a Nuclear Energy Advisory Committee, which started looking at the needs to make a recommendation to DOE. Pasamehmetoglu also asked industries that were involved in test reactors whether a test reactor was needed and would be used. They identified the type of testing they wanted to see. They made presentations to the DOE and there was quite a bit of congressional support. INL and DOE are not allowed to lobby, but if Congress shows an interest, who hears it from industry and the Nuclear Energy Institute (NEI), they call INL and ask for an explanation of the program. The possibility of faster testing in the industry and U.S. losing the leadership role in the industry are points that bring Congress to support the program. The biggest challenge is always budget.
Q6 - Design of the Versatile Test Reactor
Bret Kugelmass: What is the order of operations for designing a reactor?
Kemal Pasamehmetoglu: Reactor design starts from the fuel. First, you decide what fuel you want to use. INL decided to go with metallic fuels for their test reactor. Then, they started assembling that in a computer to determine the geometric layout of the reactor and how big it needs to be to maintain the functional requirements for flux and height in order to complete the experiments. The INL Versatile Test Reactor will not generate electricity, so they don’t need to worry about commercialization; heat just needs to be removed from the system. At this time, they are looking at something on the order of a 250-300 MW reactor. They wanted to keep it as small as possible, for cost and ease of operation, in terms of refueling and executing experiments. Metallic fuel was chosen because it makes it a bit smaller volume, but also because of the U.S. experience with metallic fuels in the 80’s and 90’s. Metallic fuels offers certain advantages compared to ceramic fuels, in terms of feedback effects, primarily temperature. The Experimental Breeder Reactor (EBR) utilized metallic fuel and sodium coolant. INL’s primary coolant will be a pool of sodium coolant, but will have the capability to test other coolants. An experimental vehicle could be placed in the same geometric cavities where fuel rods are removed. The EBR’s safety experiment was to shut down the pumps, but not the reactor, so the sodium is no longer circulating. Fuel starts heating up, causing the reactor to shut down because of negative reactivity feedback as a result of the heating up of the fuel and as the fuel expands, the neutrons escape, moderating the reaction.
Q7 - Integrated Energy Future
Bret Kugelmass: Where is the future of the nuclear industry going?
Kemal Pasamehmetoglu: People will come to realize nuclear energy is needed. We will not be well served if we try to solve the entire problem with one technology, because there is competition for limited dollars with other technologies. The problem should be looked at as an integrated energy problem and look at different energy sources in areas where they do the best and not make it a competition. Each technology can be used at their best so the sum of the whole is greater than the sum of the parts. Smaller, more flexible reactors and advanced reactors can be integrated with other systems and operate with more flexibility.
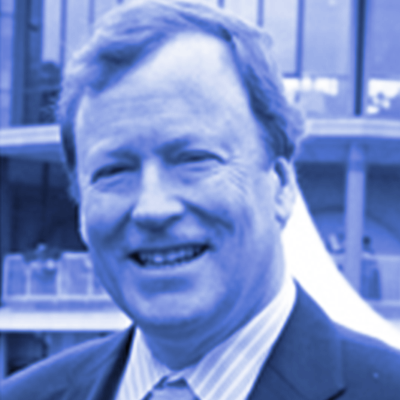
1) David’s personal journey through the nuclear industry from executive vice president of NAC International to president of the US Nuclear Industry Council
2) The history of energy in the US including the Advanced Reactor Program and why it went under
3) The Nuclear Industry Council’s two main focuses of advanced technology and supply chain and the significance of each
4) David’s outlook on the US nuclear industry including potential non-light water reactor startups microreactors at the Department of Defense complex
Q1 - Entrance into the Energy Sector
Bret Kugelmass: Where did you grow up?
David Blee: David Blee was born in the remnants of the British Empire in Karachi, Pakistan, as a son of a foreign service officer. His family then moved to South Africa, and then spent 6 years during his formative years in India. After Blee moved to Washington, D.C., he attended college in Pennsylvania and cut his teeth in political campaigns on Capitol Hill. His last stint on Capitol Hill was as chief of staff to Congressman Connie Mack, who later went on to be a Senator in Florida. As he was coming off a campaign, Blee was given an appointment through the White House as a director of public affairs to the Department of Energy (DOE). He later went on to head up director of external affairs where he was in charge of congressional and public affairs. At this point, Blee was completely immersed in energy; one of his first issues in the nuclear energy space was Yucca Mountain. Blee was drawn to the energy sector because he recognized early on that it was very important to everyday life, as well as the economy and national security. Blee also dealt with natural gas electricity market deregulation and concerns about nuclear plant shutdowns.
Q2 - Management of Depleted Uranium
Bret Kugelmass: What was it like during the early talks of advanced reactors?
David Blee: Twenty years ago, talk of advanced reactors was very government and National Lab centric, creating a downselect environment where one design is selected, as opposed to todays’ entrepreneurialism. At the point, the administration was unsure about its commitment and advanced reactors basically disappeared. At one time, there were 57 reactors at Idaho National Labs. David Blee took the plunge into nuclear energy full time when he became executive vice president of NAC International, a technology and services company. The front end group was focused on the whole fuel cycle. The technology side was focused on the back end of the system and the technology systems that manage spent fuel. Spent fuel was previously stored in pools to cool off, but eventually decided to re-rack the systems, since the government was supposed to pick up the spent fuel in 1998 under contract. The at-reactor storage outside spawned that industry. Nuclear fuel is not a gas or liquid, but is the form of a rod. Companies sued the government for storage costs that they shouldn’t be paying for and now the government is paying $5 billion a year for on-site storage. Most folks also didn’t know they were signing up for 100 year storage at their sites. Maine Yankee is an orphan site, which has already been decommissioned and has no others nearby. It is empty except for 64 canisters sitting in storage and the site must be maintained and secure, paid with government money. A repository is needed at the end of the day, whether you recycle the material or not. Depleted uranium still has commercial value; some estimates of the value of depleted uranium from the uranium enrichment facility in Paducah is worth $5 billion dollars. Yucca Mountain, interim storage, and technology should be pursued to address spent fuel.
Q3 - United States Nuclear Industry Council
Bret Kugelmass: Can we invest in technologies to reduce or eliminate nuclear waste instead of invested so heavily in Yucca Mountain?
David Blee: David Blee believes in closing the nuclear fuel cycle to reduce or eliminate nuclear waste. There are currently 70,000 metric tons of spent fuel in the U.S., which could be placed in canisters and stored on a plot of land 40 acres in size. The U.S. is produced 2,000 tons each year from the reactor fleet. It will take 50 years to ship all the spent fuel to a central location. Consolidated storage with staging grounds, a reactor, and recycling could benefit the reduction of inventories and put them to use. The U.S. Nuclear Industry Council is a business consortium focused on opportunities in advanced nuclear and global markets from a hybrid supply chain perspective. The group started with a back end fuel cycle focus when it looked like Yucca Mountain was going to get going in the early 2000’s. In 2009, the Obama administration terminated the Yucca Mountain program, but the general consensus of the group was that nuclear remained important. There was a lot of competitive energy in the U.S. because of cheap gas, and Fukushima also affected the nuclear renaissance. Blee’s group decided to expand the focus on the resurgence of American nuclear energy and propelling technology to extend the current fleet and support innovation. The supply chain must be ready for nuclear and needs to stay the best in the world. The U.S. had atrophied in terms of their ability to construct big reactors, only constructing 65% of the big reactors domestically since the U.S. lost its way in manufacturing. Small reactors will be the nuclear supply chain renaissance as they will be factory assembled.
Q4 - International Nuclear Market
Bret Kugelmass: Will we have trouble competing in international nuclear?
David Blee: Advanced technology is the game changer and market disruptor for international nuclear. The AP-1000 pressurized water reactor (PWR) is the best big reactor, featuring passive safety features. China, Korea, Russia, and France are all pushing enhanced nuclear designs. The U.S. is still using the same technology the U.S. Navy decided they wanted to use years ago for their ships, submarines, and aircraft carriers. There were other designs that were more innovative that were less appealing to the Navy. The Russians are using a build on operating approach, where they come in and build it, take a 49% share, operate it, co-own it, and bring all the project financing. U.S. energy companies have to go to market where they must win on best value. The U.S. needs to remain the go-to country for nuclear energy, meaning advanced reactor innovation and a versatile test reactor at Idaho National Lab. Other countries want the American supply chain because it runs the best plants in the world, operates the best safety culture, and has the deepest and widest supply chain. Power companies’ first focus is job one, which is the current fleet and revenue. This is a big disadvantage to the international market, since the utilities do not usually go to the international market.
Q5 - Nuclear Program Financing Challenges
Bret Kugelmass: What needs to change in the U.S. to keep competing more effectively from a financing perspective?
David Blee: Financing across the board, in terms of venture capital money, has at least a ten to fifteen year payoff. The Export-Import (EXIM) Bank needs to get back in action. A prerequisite for any bid is whether you have an export-import bank that provides financing. EXIM bank right now is not fully staffed up right now due to political reasons. The last Congress tried to kill the EXIM bank, but the President has come around in terms of recognizing the return on investment. Getting OPEC, the Overseas Private Investment Corporation, involved is also important and they are redlining nuclear projects right now. There will still be a desire for big plants globally, such as a six-pack in India and an eight-pack in China. The real game changer to the financing issue is to have small reactors, which are maybe $1-3 billion dollars which is easier to acquire funding for. As a rule of thumb, when you start to get volume orders, there is a 20% reduction in cost. Big plants, in some respects, are one-offs in the sense of assembly at the site and not being as scalable. Fleet extension is important, but the new plants should be able to run 100 years. Wind and solar are only going to run 20-25 years and are treated the same as nuclear, even though the asset will be there for 80-100 years. Getting a loan guarantee program back on track is helpful to both state regulators and the finance community. People should go see a nuclear plant and go inside to see how it operates. A relatively small footprint produces the same as thousands of windmills or miles of solar collectors. Nuclear needs to be socialized and demystified to break the code to the financial community.
Q6 - Future of Nuclear
Bret Kugelmass: What are some of the more global forces at play and where do we see new nuclear markets emerging?
David Blee: New nuclear markets are emerging in Asia. Right now, there are roughly 60 plants being constructed around the world and 150 more on the drawing board. 1.2 billion people currently don’t have electricity. Electric vehicles is electrification of the transportation sector. Saudi Arabia will need massive amounts of electrification for their water, in terms of driving their desalination plants. Vietnam, Malaysia, and Indonesia are still looking to realize the value of nuclear energy. There is a lot of desire for self-sufficiency, and having baseload nuclear energy gives a lot of confidence for your economy 24/7. David Blee is very optimistic about the future of nuclear and is encouraged to see the advanced nuclear community mobilizing, as well as a desire to be the world leader in the supply chain. People want clean energy. The pipeline must be filled for the 2020’s and Vogtle must be completed. There is potential for small modular reactor (SMR) deployment and completion of Bellefonte plant in Alabama. Replacement for the existing nuclear fleet will happen in the 2030’s. Nuclear is a dedicated industry and the people are committed to putting their heads together when facing a challenge.
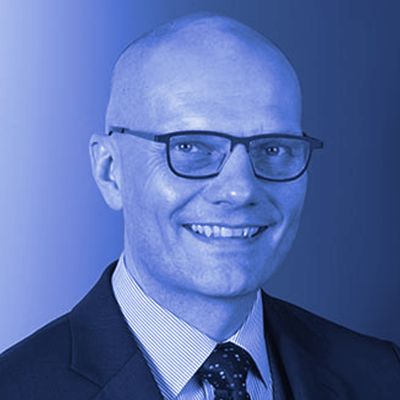
1) Paul’s personal evolution in the nuclear industry working at Capehurst, Berkeley, and now at the NNL
2) A deep dive into nuclear fusion technology
3) Political, financial, and technological challenges the industry must overcome to secure nuclear as our primary energy source
4) Improving communications strategies surrounding nuclear safety to increase trust and public discourse
Q1 - History of Nuclear in Manchester
Bret Kugelmass: What is the National Nuclear Laboratory?
Paul Howarth: The National Nuclear Laboratory is owned by the U.K. Government and what remains is the U.K.’s strategic nuclear technical capability that resides within the public sector. U.K.’s nuclear industry went through significant change about ten years ago that led to the establishment of the laboratory, which now operates with about 1,000 people. The lab does not receive any grants directly from the government, but is funded purely from the work they do in the commercial sector. Generated profit is recycled back into long term skills research and development for the future of U.K.’s nuclear program. Paul Howarth grew up just South of Manchester and attended school there. The Industrial Revolution started in Manchester and is home to the original steam engine. Through the cotton trade, Manchester also had strong ties with the U.S. and the Caribbean, but took a strong stance to abolish slavery taking place. Steam power generation was started for the mills for the cotton industry. Rutherford also split the atom in Manchester in the 1900’s, and there are more than ten nuclear Nobel prizes that have been won in Manchester. Because of the richness that Manchester got through the Industrial Revolution, the importance of universities was recognized, leading to a world leading university. In the early days of nuclear, Cambridge University set up the Cavendish Laboratory and Manchester wanted to rival it with world class laboratories that would attract world class people.
Q2 - Advanced Nuclear Technology
Bret Kugelmass: What sent you down the path to astrophysics?
Paul Howarth: Paul Howarth was attracted to astrophysics from the point of view of how to explain the universe from the point of view of mathematics. After completing those studies, Howarth felt a drive to do something which benefited people and was important to a lot of people. He got involved in nuclear fusion technology, completing his PhD on the topic while in Oxford at the Joint European Torus project. During Howarth’s first week, the project was doing the world’s first deuterium tritium experiment. His PhD was based on nuclear technologies and how to measure what is coming off the plasma. Paul Howarth was approached by British Nuclear Fuels (BNF) and invited him to become part of their new company at Sellafield. While Howarth was at Sellafield, he was invited to work on technology transfers in plasma in Japan. Howarth knew BNF’s biggest customer is Japan and Sellafield used to reprocess nuclear fuel for the Japanese utilities. Howarth originally spent 12 months in Japan, but extended his stay to about two and a half years, spent at different locations such as Fukuoka on the semiconductor industry at the Tokaimura nuclear reprocessing plants. Japan has no indigineous oil and gas and knew they had to look at nuclear technology. If not for Fukushima, Japan may have been wedded to a closed fuel cycle strategy. Paul Howarth returned to the U.K. working for British Nuclear Fuels to look at advanced technologies. Howarth also spent some time running operations at Berkeley Power Station. In 2003, Howarth joined a small group in BNF that was working to build the case for new nuclear, mainly engaging with government to get them to recognize the importance of a new nuclear build program.
Q3 - Nuclear Energy Policy in the U.K.
Bret Kugelmass: Tell me about U.K. energy policy.
Paul Howarth: In 2002, the U.K. published a white paper committing to a 60% reduction in CO2 emissions through renewable technology. One chapter was written for nuclear, but was taken out at the last minute. At the time, British Energy, which ran the U.K. reactors before it was EDF Energy, bombed and the business model failed. The government had to bring the reactors back into government ownership. This failure had nothing to do with the nuclear fleet, but instead that the company hedged wrongly and sold electricity wholesale as opposed to retail. Paul Howarth, with his small team at British Nuclear Fuels, started working with government to try and build the case for nuclear by addressing myths about safety and reliability. This group worked with industry, the general public, and the House of Lords. When Putin cut the gas supplies to Ukraine, the U.K. government realized energy was not a pure commodity and energy security is important. This changed the U.K. government’s perspective on nuclear. One mistake made in the past was for Sizewell B, which went through a local planning inquiry to deconstruct the technology and make engineering decisions. Howarth’s group told the U.K. government that clarity on the waste management policy was needed, as well as certainty of the planning process, which led to the generic design assessment process, and certainty over the contracts for electricity. The public was not given a choice to say no to nuclear without providing an alternative for meeting carbon goals. There was recognition of the importance of nuclear during this consultation and the public wanted to proceed with the nuclear projects.
Q4 - Rebuilding U.K.’s Nuclear Programs
Bret Kugelmass: Tell me about setting boundaries between the experts and the public.
Paul Howarth: After the consultation was launched, Paul Howarth was concerned with U.K.’s strategic technical capability. The government said they would support nuclear, but would not subsidize the technology, which led to a lack of investment in nuclear R&D and nuclear skills. Howarth and his group worked with Manchester University, at a time in which nuclear is rock bottom, to build up technical skill. Birmingham University had a well-known master’s program in physics and technology of nuclear reactors, but at one point only had five individuals in the program. Afraid that the U.K. would lose its nuclear capability, Howarth and a colleague worked with BNFL to set up the Dalton Nuclear Institute at the University of Manchester. The nuclear industry has a lot of bright individuals, but also a lot of people with passion. Nuclear power is an energy source that is going to support the future of the planet.
Q5 - National Nuclear Laboratory
Bret Kugelmass: How did you join the National Nuclear Laboratory (NNL)?
Paul Howarth: When the nuclear industry was broken up, the U.K. had BNFL’s science and technology division. A national laboratory was set up in a GOCO contract, modeled after the national labs in the U.S. A consortium was formed between Batelle and Serco and won the bid for the lab, where Paul Howarth became part of the management team. In 2011, Howarth took over as chief executive of the National Nuclear Laboratory (NNL). In the U.S., labs are funded by the government. In the U.K., the lab is commercially funded. The NNL delivers to time cost of quality work for their customers, incentivizing the workforce to bring in more profit which goes back into technical skills training and development. Paul Howarth worked with Battelle and collaborated closely with the labs in the U.S. Howarth’s position at the national lab puts him in a position between industry and academia, allowing him to understand the differences between the cultures of the different organizations. Because of Brexit, the government has been looking at its industrial strategy and which are big enough to provide a comfort blanket, one of which is nuclear. Nuclear is going to underpin the economy, energy, defense, and regional economy. The cost of nuclear must go down by working towards fleet build. Innovation will play its part and help with future reactor systems, but the here and now must be building these reactors with confidence.
Q6 - Innovations in the Nuclear Sector
Bret Kugelmass: What are some innovations needed in nuclear?
Paul Howarth: Paul Howarth does not see engagement between nuclear and other industries to push innovation in finance, project management, safety cases, and other areas. The U.K. has the innovation association for nuclear reactors, but innovation is also needed to deliver waste management and clean up the related challenges. Nuclear in the U.K. has always had a close association with its origin in a military program, which the opponents of nuclear turn to. Within the general public of the U.K., safety is not a concern for nuclear. The challenge with the general public is the expense and the waste. During the oil crisis of the 60’s and 70’s, the vendors of the reactor systems looked at advanced technology and recognized they need to get nuclear efficient, increasing the size of the reactors. However, this increases the capital cost and construction schedule, adding that cost back in. Gas-fired and fossil fuel plants have an unknown fuel cost at the point of construction. Nuclear, however, has a massive up front capital cost, but a very predictable and insignificant fuel cost for the next fifty years. If not many reactors are built, the weighted average cost of capital goes up and dominates the levelized cost of electricity.
Q7 - Energy Mix Model
Bret Kugelmass: Why not shrink the reactor size?
Paul Howarth: If more nuclear reactors are built, confidence in the build will increase and novel and innovative manufacturing techniques can be put into practice. Risk is decreased when confidence is increased. Building lots of reactors reduces the levelized cost of electricity generation, which allows you to build more reactors. The U.K. has a program of generation III reactors, which allows the government to look at novel technology and address the nuclear economic dilemma. Nuclear is important to Paul Howarth. At one meeting, the chief government scientist was done to look at the whole energy ecosystem. The energy challenge is so big that we need every tool in the toolbox, including renewables. A user-friendly calculator model for the U.K. general public provides a tool to play with the energy mix to solve the energy problem virtually. If the model is run without nukes and a goal of hitting 80% reduction in CO2 emissions and other factors, the model would not close and could not get the goal. After ramping up renewables and biomass, the slider moved on the model, but the model still struggled. After ramping up wave and tidal, the model still wouldn’t close. Wind power and wind farms were added, domestic heating was decreased in the winter to 12 degrees, and they couldn’t get the model close. Once they ran the nukes, the model closes. The U.K. grid is around 80 GW in size. Because the transportation sector must be electrified, a grid four times the size of the current is needed, approximately 300 GW. The model settled at a point which was 100 GW nuclear, 100 GW renewables, and 100 GW fossil fuels with sequestration. However, the U.K. currently only has around 5 GW of renewable energy, but with load factors, it is currently equivalent to about 1 GW. Also, nobody has sequestered any CO2 from any fossil fuel plant successfully at a large industrial scale. When Paul Howarth looks within the nuclear industry, he considers the meeting reviewing the energy model and feels confidence in what he is doing with nuclear.
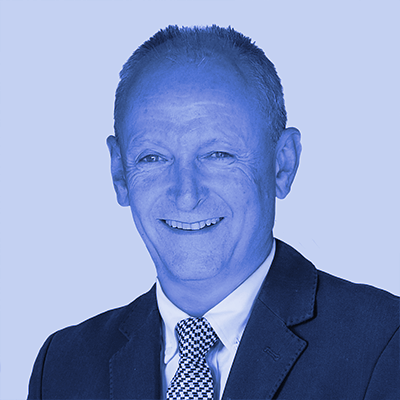
1) Ian’s background in biology and personal evolution through the nuclear industry
2) Why molten salt reactors are safer and thus cheaper when it comes to materials and construction
3) The life cycle of molten salt reactors
4) Ian’s positive outlook on nuclear’s future and solving its two key problems of cost and energy storage
Q1 - Entrance into Nuclear
Bret Kugelmass: How did you start out in the pharmaceutical and biology industry?
Ian Scott: Ian Scott’s first career was with Unilever, where he became one of four chief scientists for the company. Scott left in 2003 because he wanted to go back to the lab and do real experiments instead of managing. This took Scott into the biotech field and to a company called Synergy Biosystems, which looked at high order synergies among biologically active ingredients. After ten years, Scott retired shortly before seeing an article saying that EDF Energy in the UK were going to be paid nine and a half pence per kilowatt hour from Hinkley Point C nuclear power station. Scott became interested in why nuclear had gotten ludicrously expensive. Scott’s intention at university in Cambridge was to study particle physics because it looked fascinating to him. During his first year, scientists basically solved particle physics with the standard model. There were gaps, including Higgs Boson, but Scott saw that the next forty years would just be filling in the gaps.
Q2 - The Economics of Molten Salt Reactors
Bret Kugelmass: What did you see in nuclear in terms of the electricity problem?
Ian Scott: Ian Scott saw nuclear energy as an economic problem for how it would fit into the electricity space. Nuclear fission itself is simple, but the challenge is how to do it economically. In the early 1970’s, the price per kilowatt of building a nuclear power station was cheaper than the price of building a coal-fired power station. Nuclear made economic sense, until Chernobyl, Fukushima, and Three Mile Island happened. The early, cheap reactors were great until they weren’t. The industry fixed the problems, but at a high cost and priced nuclear out of the market. Pressurized water reactors are government vanity projects and are not market driven. Scott reviewed every concept for nuclear reactor that had been published and saw reasons why every single one would never crack the economic problem, such as a major engineering issue that would pile cost into the design. The one thing that caught Scott’s eye was molten salt fuel. Uranium can be used just as a metal, or it can be turned into a compound, such as uranium oxide, to form a ceramic, which is used in most light water reactors. Uranium oxide holds the fission products in the form of a very high pressure gas, which is dangerous if released. Molten salt sticks to uranium and turns to a different chemical, chloride or fluoride. These are salts and at the temperature a reactor operates are molten and the fission products, cesium and iodine, are no longer gases. If the containment of the molten salt fuel is breached and a hole is knocked in the concrete containment of the reactor pressure vessel, there will not get a serious reading of radioactivity at the boundary of the site. There is an intrinsic safety to molten salt fuel. One of these reactors was made operational at Oak Ridge National Lab in the U.S., the Molten Salt Breeder Reactor. Molten salt fuel must be pumped around and reactors create extremely radioactive spent fuel, which is dangerous if there is a leak. The key insight leading to Moltex Energy was that the whole paradigm of having to pump the molten salt around was false, but the salt fuel can instead be put in tubes in fuel assemblies in the reactor core and removed when they are burned up.
Q3 - Molten Salt Reactor Coolant Systems
Bret Kugelmass: What kind of coolant is used in a molten salt reactor?
Ian Scott: Molten salt coolant, without any fission products, is used in molten salt reactors. The metal tubes containing molten salt with fission products are placed in molten salt coolant. Ordinary stainless steel swells in radioactive environments, so ferritic steel is used instead, since it has good properties in a radiation field. To get the heat out of the system and create electricity, coolant is circulated throughout the core, passes it to a second molten salt coolant loop, and then goes to a third molten salt loop, which has solar salt. The third loop is used to get it to large tanks, which double as a storage system to allow for grid flexibility. Battery storage is still too expensive and it has significant carbon and waste footprints. Electricity cannot be stored at a large scale economically, but heat can be stored in solar salt. Being able to dispatch nuclear power when the renewables are off and the demand is high makes a big difference in economics and capital costs. A 300 MW nuclear reactor with a large storage system could be paired with a 900 MW steam turbine power station. The total plant cost per kilowatt electricity, including storage and turbines, goes down a lot because the cost of the nuclear plant is being divided among three times as much power as its producing. Steam systems have to be inside a containment because they are radioactive and highly pressurized. This containment is usually a meter thick, heavily reinforced nuclear concrete structure, which costs a fortune, and contains the steam generators, heat exchangers, and depressurizers. By taking the steam system away from the reactor, it is not a nuclear regulated phenomena.
Q4 - Molten Salt Reactor Life Cycle
Bret Kugelmass: Why don’t pressure water reactors add another heat transfer loop of some sort of salt and move everything out of the containment dome?
Ian Scott: Pressure water reactors run at low temperatures, with an output temperature of about 300 degrees centigrade. The temperature must be around 600 degrees for the heat storage to work. Ian Scott’s molten salt system is different than other molten salt systems because of the differences in pumping. For salts to be pumped, a reaction chamber is needed and must last the life of the reactor. Terrestrial Energy’s concept is to throw away the whole reactor system every seven years, but there is an issue of whether you can pay for building, running, and decommissioning it based on seven years of output. Scott’s reactor is designed to last sixty years, since the bit that holds the fuel is in fuel assemblies and come out the reactor every five or ten years, just like conventional reactor fuel. Because of the intrinsic safety of molten salt reactors, there is no pressure in the core. Scott’s design uses chloride, because his reactors are fast spectrum reactors which do not have a moderator. This requires a higher amount of fissile material in the core, but also burns up the long-lived actinide which accumulates in the waste and thermal reactors. This platform technology allows for both fast and thermal reactors the use of chloride and fluoride fuels. Ian Scott uses chloride fuels because it can be made very strongly reducing to avoid corroding metals. Chemistry must be adjusted to avoid uranium metal and chromium chloride, but instead to have equilibrium of that reaction of chromium metal and uranium chloride. This is harder to do with fluoride.
Q5 - Nuclear Fuel Qualification
Bret Kugelmass: Have molten salt fuel experiments been completely modeled out?
Ian Scott: Qualification of the fuel is one of the hardest parts of developing a new reactor. For a uranium oxide fuel, it needs to be put into a reactor to see how the pellets behave when they’re being fissioned. This cylindrical pellets have lots of cracks and swell until they touch the wall of the metal cladding; if the fuel is not right, it can rupture the walls. Molten salt fuel is a liquid and has no pressures. This type of metal can be tested in two ways: if it causes corrosion of metal, and what happens to the liquid as it fissions. The things that make qualification of fuel hard don’t apply to molten salt fuel. Nuclear regulation is entirely a national responsibility and different regulators operate very different systems. The U.S. regulators went down a route for regulation in which they wrote out rules, and if the rules are passed, the reactor is safe and it will get approved. The problem is that the rules were written for pressurized water reactors and to approve a different reactor, one must produce a new set of rules. The nuclear regulators in U.K. and Canada operate similarly, which have no rules, but a case must be assembled to prove your reactor is safe. This system is better for new technology and those that have intrinsic safety principles. One of the problems with nuclear is that everyone thinks it’s the government’s job to develop new nuclear technology. Its roots and culture were in the military complex.
Q6 - Controlling Nuclear Construction Cost
Bret Kugelmass: How do you know how much your plant is going to cost?
Ian Scott: In mega project territory engineering, times two cost overrun is almost through for any sector, including nuclear reactors, bridges, and tunnels. These projects are so big and complex that they just tend to go wrong. If the reactor can be smaller, there is more predictability in terms of cost and reduces the construction period. Ian Scott’s molten salt reactor has a construction period of three and a half years. The industry as a high experience rate and competency for building turbine systems, which is approximately 60% of the construction cost. Coal-fired or oil-fired gas plants could be modified by swapping out the coal furnace with a nuclear reactor and turning it into a nuclear power station. In order to do this practically, the steam island must be functionally separated from nuclear. If the reactor shuts down, steam turbines cannot be used to get rid of decay heat. Once you make a small claim on the turbine system to keep the reactor safe, it becomes nuclear. Molten salt storage tanks allow steam turbines to be separated from the reactor. China is building a lot of coal-fired power stations because they don’t want to tank the economy, even as they want to move away from nuclear. They want to take sunk capital costs and go carbon free with a nuclear retrofit. If nuclear can be cheap and utilize stored energy, Ian Scott sees no reason by the world is anything other than wind, solar, and nuclear power. This gives a complete, robust energy system with zero fossil fuel burning. This is a future in which consumers spend less on their electricity than they do now; cheap electricity brings higher quality of living.
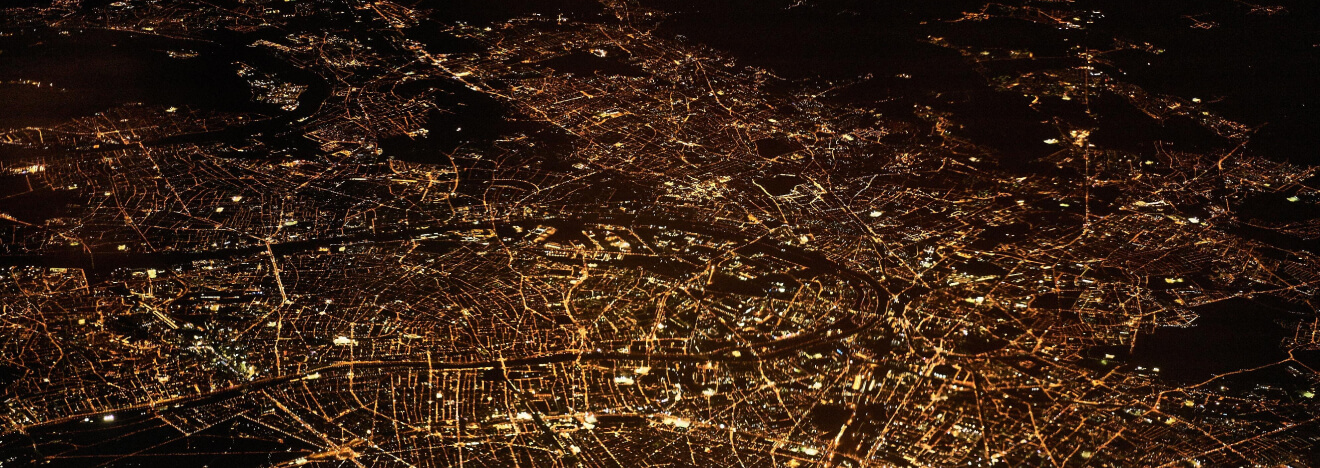
Sign up for our newsletter
No results found
Please try different keywords